Appendix 3 Genetics, fragmentation and climate change — implications for restoration of local native vegetation communities
Two primary threats and their interactions need to be recognised by revegetation practitioners. These are fragmentation and climate change.
Effect of fragmentation on genetic diversity
The concept of confining seed collection to a 'local provenance' area (to ensure local adaptation is maintained) has been widely adopted by plant-based restoration practitioners. However, the paradigm of collecting very close to the restoration site is no longer considered useful. Firstly, scientists agree that plant local adaptation is not as common as many believe. Secondly, many practitioners now understand that a 'local' genotype may occur over wider areas (i.e. from 10s to 100s of km) depending on the species and its biology. However, in a largely cleared landscape, small fragments are at risk of elevated inbreeding when populations of a species drop below threshold numbers, which can be different for every species. As inbred seed may fail to reinstate functional and adaptable plant populations, in general it is best to collect seed from larger, higher density stands. This means that in fragmented landscapes where vegetation stands are smaller, less dense and more isolated, collecting seed from wider distances and multiple sources will be necessary to capture sufficient genetic diversity to rebuild functional communities. This seed should be multiplied in regional seed production areas, however, to avoid overharvesting from remnants.
Examples of seed production areas.
Climate change
Examination of Australian ecosystems shows that many native species have endured ancestral extremes of climate well beyond predicted climate change scenarios. However, accelerated climate change is a serious emerging problem. Some species will be impaired by increasing ocean temperatures and acidity while marine, freshwater and terrestrial habitats will be lost in some locations due to sea level rise. Many river channels, lakes and wetlands may also be affected by drying or its consequences such as increased salinity—and cold-adapted species will be lost at colder, higher elevations where there is nowhere higher for them to migrate as climate warms. Indeed, even conservative global warming scenarios suggest that a wide range of local environments to which species may have adapted will change dramatically.
Box 7 Climate envelope
The climate range in which a species currently exists can be referred to as its ‘climate envelope’.
During climate change this climate envelope is likely to uncouple from the current location in which the species exists and, where conditions become hotter, move further poleward or to higher elevations. This means that the species may be lost from the more equatorial extreme of the range and need more help to adapt as it, or its genotypes, move poleward or to higher elevations.
However, as precipitation is likely to change in less predictable ways, it is likely that the displacement of climate envelopes will be more complex.
Although we cannot precisely predict the type and scale of risks that ecosystems face because only a small proportion of species has been individually studied, we know that some species may be lost from their current locations while others will colonise new areas, altering local species assemblages. We also know that the effect of climate change will be particularly strong when combined with high levels of fragmentation.
Some species may have sufficient inherent ‘adaptive plasticity’ to persist as climates change, as has been demonstrated from translocation experiments and detailed pollen analysis of past environments. That is, an individual plant may be able to adjust its form by mechanisms such as reducing its leaf size, increasing leaf thickness or altering flowering and emergence times. But in many cases, persistence may depend on a species’ capacity for genetic selection or adaptation, which in turn depends on population size and the diversity of the genes available.
Species that have large, connected populations, a wide climatic range, naturally high dispersal characteristics and whose populations have many genes in common are likely to have a higher chance of genetically adapting to the new environments or migrating as their climate envelope moves (Box 7). Conversely, species with low pollen and seed dispersal characteristics, that occur naturally in ‘islands’ or ‘outliers’ or that have been isolated through land clearing or river regulation, for example, may be less able to adapt or migrate in response to climate change.
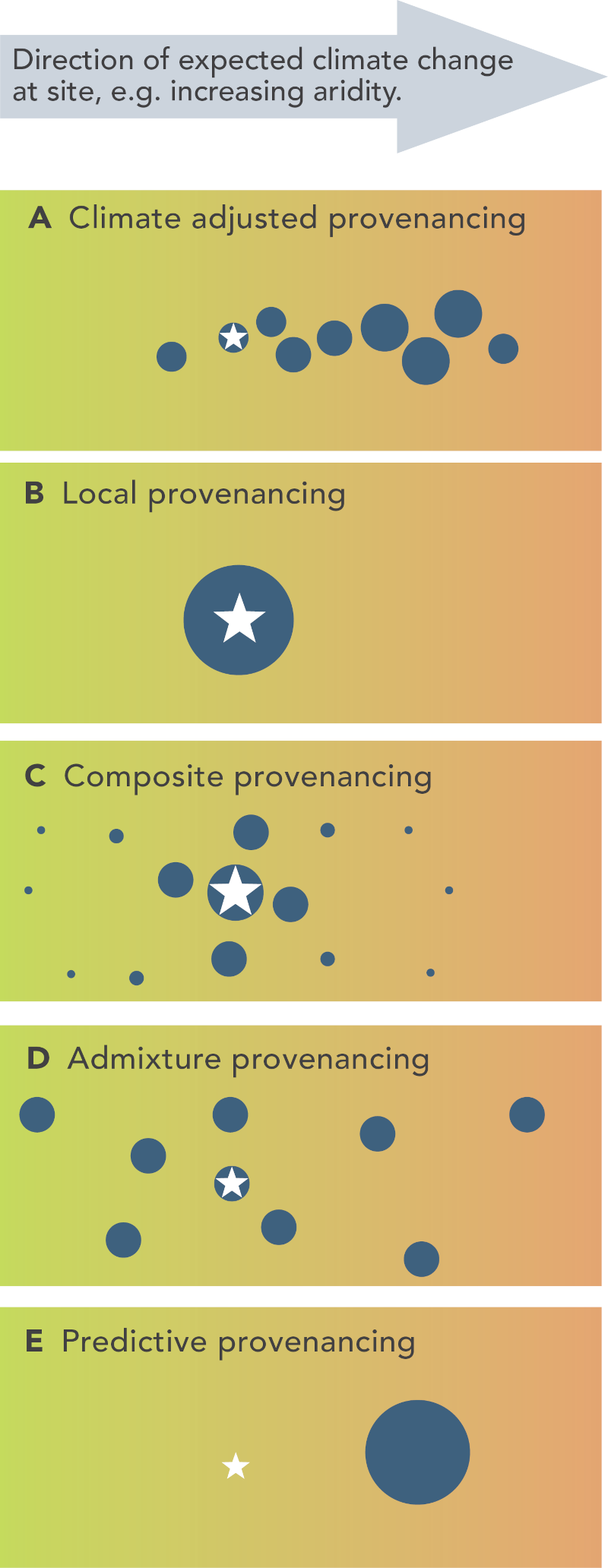
Figure 7 Provenancing strategies for revegetation, (Reproduced here from Prober et al 2015) The star indicates the site to be revegetated, and the circles represent native populations used as germplasm sources. The size of the circles indicates the relative quantities of germplasm included from each population for use at the revegetation site. In the case of the climate-adjusted provenancing the relative quantities of the germplasm from the various populations will depend upon factors such as genetic risks, and the rate and reliability of climate change projections. For simplicity this represents the major direction of climate change in a single dimension (e.g. aridity, to combine influences of increasing temperature and decreasing rainfall), but multiple dimensions could be considered as required.
Implications for restoration
Techniques and protocols are emerging to guide the collection of genetically diverse material to use in revegetation to enhance a species’ adaptive potential. In extensive, intact native habitats where species and populations are likely to have a greater capacity to adapt unaided because of high connectivity, interventions to enhance adaptive potential are unlikely to be needed. But where landscapes or waterscapes remain largely fragmented, interventions to assist genetic adaptation are expected to be beneficial. This means that, while the local gene pool still has potential to play a major role in adaptation, it is prudent to consider including at least a small amount of germplasm of the same species from a ‘future climate’—that is, a region with a climate similar to that which is predicted for the area being restored. Research is underway to test some of these new approaches and it is hoped that ‘rules of thumb’, will eventually be developed. Meanwhile, researchers are designing protocols and proformas for appropriately documented and registered ‘citizen science’ trials integrated into low risk restoration settings. Participation in such trials will enable groups to actively test a range of recommendations on their sites while also optimising opportunities for improved science and practice.
Tools for assessing climate-readiness in relation to genetics
Some tools are available to help restoration planners undertake what could be called ‘climate readiness’ analysis at the planning stage. Firstly, restoration practitioners are encouraged to seek out predictions of locations where ecosystems are likely to be affected by climate change. Secondly, practitioners are encouraged to liaise with researchers to gain a better understanding of predicted responses of species to both fragmentation and climate change and to identify the relative risks of a range of options relating to the deliberately movement of genetic material in restoration projects. (Genetic analysis can be undertaken by a range of research institutions and is increasingly affordable for practitioners. This cost reduction is increasing numbers of species being studied while rapid improvements in the effectiveness and efficiency of genetic testing tools is also occurring.)
Web-based tools are also readily accessible for identifying whether the species currently occurring in the vicinity of your site will still be suited to climates predicted to occur at your site in the future. One of the most important of these is the Atlas of Living Australia website (www.ala.org.au) which can help practitioners identify the natural geographic range of a species and whether it may have potential to tolerate the conditions predicted to occur under climate change scenarios which themselves are mapped on the website www.climatechangeinaustralia.gov.au. An explanation of how these tools can be combined is found in Booth et al. (2012).
Proposed propagule sourcing strategies to build climate-readiness into restoration through ensuring genetic diversity include: composite provenancing (Broadhurst et al. 2008), admixture provenancing (Breed et al. 2013), predictive provenancing (e.g. Crowe & Parker 2008), and climate adjusted provenancing (Prober et al. 2015, Fig 7). Application of any such models should be undertaken within a risk management framework that considers the potential negative effects of inbreeding and outbreeding depression, interpreted in a manner clearly understood by practitioners. It should also include long- term monitoring (i.e. at least a decade) to enable lessons learned to be captured for both restoration and climate science.
Practitioners designing planting lists need to bear in mind, however, that it is impossible to be certain of the changes that will occur. Different species will respond to climate change in different ways and at the moment there is no easy way to predict this. Furthermore, temperature and rainfall are not the only important predictors. A range of physical (e.g. soils) and biological factors (e.g. dispersal)—which themselves may or may not be affected by a changing climate—can also have important roles in influencing the distribution of a species. While some caution will always be required, a balanced approach in fragmented areas would see the restoration plan specify the use of locally occurring species (preferring germplasm from larger populations, even if somewhat more distant) and where advised, formally trialling the inclusion of some germplasm from ‘future climate’ locations. Such a combined approach—coupled with optimising connectivity to the extent possible—is likely to improved opportunities for natural adaptation should it be required.
References
Booth T.H., Williams K.J. and Belbin L. (2012) Developing biodiverse plantings suitable for changing climatic conditions 2: Using the Atlas of Living Australia. Ecological Management & Restoration 13:3, 274- 281.
Breed M.F., Steed M.G., Otewell K.M., Gardner M.G. and Lowe A.J. (2013) Which provenance and where? Seed sourcing strategies for revegetation in a changing environment. Conserv. Genet. 14, 1-10.
Broadhurst L.M., Lowe A.J., Coates D.J., Cunningham S.A, McDonald M., Vesk P.A. and Yates C.J., (2008) Seed supply for broadscale restoration: maximizing evolutionary potential. Evol. Appl. 1, 587-597.
Crowe K.A. and Parker W.H. (2008) Using portfolio theory to guide reforestation and restoration under climate change scenarios. Climatic Change 89, 355-370.
Prober SM, Byrne M, McLean EH, Steane DA, Potts BM, Vaillancourt RE, Stock WD (2015) Climate-adjusted provenancing: a strategy for climate-resilient ecological restoration. Frontiers in Ecology and Evolution 3, Article 65.